This is the second in a four-blog series on epigenetics. In this blog, I discuss how epigenetics works. In the third blog, I will talk about a form of epigenetics referred to as behavioral epigenetics. In the fourth, and last blog in this epigenetic series, I will talk about ways to reverse negative epigenetic changes.
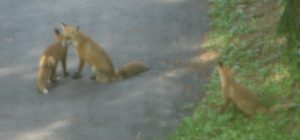
Epigenetics is a journey – where do you want to go?
In the last blog I said that “epigenetics explains how and why environmental factors can influence how our genes, formed over millions of years, can be silenced or activated to help us adapt to environmental changes that occur in less than a lifetime.” This ability of our bodies to adapt to environmental changes that occur in a matter of days or a few years, enables us to thrive in climates from the hot and humid areas of Africa, to the cold and snowy areas of Europe.
But you may ask yourself how is this possible?
To answer that question, I begin by noting that our genes are made up of DNA. DNA is the code our body uses to build and rebuild itself and consists of four basic chemicals that are strung along the double helix like beads on a necklace. But our genes need to receive instructions that tell them what to do. Our epigenome provides those instructions. If we liken our genes to computer hardware, then our epigenome can be likened to computer software that tells our genes when, where and how they should work. Telling a gene what to do turns it on (expresses it) or turns it off (silences it). This allows the epigenome to alter how our genes express themselves without changing the DNA code. This can determine what an organism looks like and how it behaves. Hence, although all of an organism’s cells have the same genome, the cells take on different functions based on the instructions given to their genes.
A gene expresses itself by making a copy of itself and sending the copy out into the cell to produce the protein encoded in its DNA. The protein, either alone or in combination with other proteins, produce a specific organism characteristic. If the gene is blocked from producing the protein, the organism characteristic will not appear. Hence, a trait encoded in the DNA of a gene like the “agouti” gene in the mice with identical genes discussed in the last blog, can have different coat colors and weight distributions depending on whether the agouti gene is expressed or silenced. As humans, most of us have genes that suppress tumor formation. However, if those genes are epigenetically silenced, our anti-cancer defenses are weak. Similarly, some of us have a gene that causes schizophrenia, which if epigenetically silenced, won’t cause us to develop that illness.
Our epigenetic software begins working when an egg is fertilized. It does this by telling our cells which should become brain cells, skin cells, heart cells, and the other specialized cells that make up our bodies. Our epigenome does this by using epigenetic modifications called “tags” that provide the instructions that tell our genes whether to be turned on or turned off. Several of these tags have been discovered. The two that have been researched most thoroughly are methyl groups and histones.
These tags work together like a dimmer light switch. Like a light switch, our methyl groups turn genes on and off. Our histones determine whether our genes are expressed a lot or a little. Our DNA is wrapped around our histones, which change how tightly or loosely our DNA molecule is wrapped around them. Like a dimmer switch controlling how bright a light is, when our DNA is loosely wound around a histone our genes express more, and when they are tightly wound our genes express less.
Epigenetics means that every cell in our body has a distinct methylation and histone pattern that tells a gene whether or not to express itself, and how much expression should occur. Some estimates are that we have four million of these switches that are triggered by lifestyle and environmental factors.
The study of human identical twins has given researchers a unique window into epigenetic changes. Human identical twins have the exact same DNA in their cells. However, many of us have met identical twins who look and behave slightly differently, depending on the epigenetic alterations that have occurred during their lives. This is because research shows that as identical twins age, especially ones that spend the most time apart, their gene expression becomes less alike. In the picture on the right, taken from a NOVA Science Now video, the areas in yellow show epigenetic areas that are identical. The top image shows the epigenetic differences between six-year-old identical twins, while the bottom image shows 66-year-old identical twins.
As noted in the last blog, an environmental factor that affects our gene expression is stress. It reshapes our brain by causing the release of a hormone, called cortisol, that is essential to our “fight, freeze, or flight” response, and critical to a child’s healthy development. When children are exposed to multiple, chronic stresses, (neglect, abuse, maternal depression, parental discord, crime and other domestic dysfunctions), cortisol levels rise and stay high.
Too much cortisol changes two parts of the brain. One is our prefrontal lobe in the front of our brain, which handles executive functions like negotiating with people, telling the difference between good and bad, and thinking about the consequences of our actions. The other is the hippocampus, deeper in the brain, which is central to creating memories of fact that are important for success in school and work.
Early-life stress turns on genes that overreact to stress, and turns off genes that help us by buffering us from the effects of stress. Hence, stress can cause epigenetic changes in our brains that can increase our chances of mental challenges like depression. Similarly, adult mice exposed to highly aggressive neighbors become socially avoidant, defeated, and subordinate, and develop histone changes in a depression-related gene.
In humans, meditation has been found to reduce the levels of pro-inflammatory genes, which in turn correlate with faster physical recovery from a stressful situation. And, a Swedish study explored the epigenetic changes resulting from endurance training of a group of volunteers who trained one randomly chosen leg, four times a week for three months. This study produced clear differences between the legs. It showed that three months of training produced significant differences between the legs in about 4000 genes, with exercise increasing activity in genes related to muscle adaptation and carbohydrate metabolism, and decreasing activity in genes related to inflammation.
At a personal level, in November 2018, the doctors treating me after I collapsed at a mall, told my wife to call my sister and children together to say goodbye because it took 45 minutes before the doctors could get my heart to beat on its own, causing them to assume I had suffered brain damage. A year later, as I focus on epigenetics and look back at my recovery, I am reminded that as the neuropsychologist, who evaluated my condition earlier this year over an eight-month period, said, after completing her evaluation of me, “I don’t know how you are.” Meaning, current medicine cannot explain my recovery. An opinion echoed by many of the other physicians familiar with my case.
My own theory for why I recovered is based on circumstantial evidence that points to the possibility of epigenetic changes that enabled me to recover from events that the doctors treating me thought would damage my brain. I begin with my freshmen year in college, when I went to donate blood, and learned that my ‘at rest’ pulse was 45 beats per minute due to my rowing crew. And six years ago, I learned, while in the hospital for observation, that my asleep pulse would drop into the twenties, possibly due to over 40 years of meditating. What is more, I learned from the nurses who kept waking me up in the middle of the night, that I was an unusual patient. They told me that most patients, when awakened with a pulse in the twenties, were groggy and disoriented, whereas I was immediately alert and focused. These events cause me to wonder, whether just as chronic stress can change our brains for the worse, exercise and meditation could have changed my brain so I could function at oxygen levels that were so low that they would cause most people to suffer brain damage.
While more research is needed to answer my wonderment, I can only be thankful that what we do in the past can help our chances of surviving life-threatening events.
In my next blog, I will talk about a form of epigenetics referred to as behavioral epigenetics.